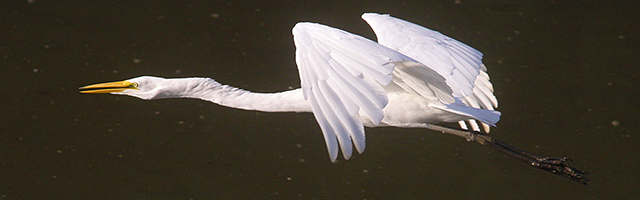
Zoology Faculty Work
Title
ACE2 is required for daughter cell-specific G1 delay in Saccharomyces cerevisiae
Document Type
Article
Publication Date
2003
Publication Title
Proceedings of the National Academy of Sciences
Volume Number
100
Issue Number
18
DOI
10.1073/pnas.1833999100
Abstract
Saccharomyces cerevisiae cells reproduce by budding to yield a mother cell and a smaller daughter cell. Although both mother and daughter begin G1 simultaneously, the mother cell progresses through G1 more rapidly. Daughter cell G1 delay has long been thought to be due to a requirement for attaining a certain critical cell size before passing the commitment point in the cell cycle known as START. We present an alternative model in which the daughter cell-specific Ace2 transcription factor delays G1 in daughter cells. Deletion of ACE2 produces daughter cells that proceed through G1 at the same rate as mother cells, whereas a mutant Ace2 protein that is not restricted to daughter cells delays G1 equally in both mothers and daughters. The differential in G1 length between mothers and daughters requires the Cln3 G1 cyclin, and CLN3-GFP reporter expression is reduced in daughters in an ACE2-dependent manner. Specific daughter delay elements in the CLN3 promoter are required for normal daughter G1 delay, and these elements bind to an unidentified 127-kDa protein. This DNA-binding activity is enhanced by deletion of ACE2. These results support a model in which daughter cell G1 delay is determined not by cell size but by an intrinsic property of the daughter cell generated by asymmetric cell division. In the budding yeast Saccharomyces cerevisiae, mother and daughter cells spend different lengths of time in G1 before entering S phase. The larger mother cell progresses through G1 relatively quickly, whereas the smaller daughter cell is delayed in G1. The Cln3 G1 cyclin promotes progression through G1 (1, 2). Multiple signal transduction pathways that regulate the rate of G1 progression converge to regulate Cln3 transcription, translation, stability, and activity. Mating pheromone and osmotic shock arrest cells in G1 in part by decreasing the activity of the Cln3/Cdc28 kinase complex (3-5). A leaky scanning mechanism involving a short upstream ORF (6) makes Cln3 translation extremely sensitive to the rate of protein synthesis: a 50% decrease in protein synthesis produces a 10-fold fall in Cln3 protein levels (7). This mechanism probably accounts for regulation of Cln3 translation by the Tor and protein kinase A pathways (7, 8). Nitrogen starvation also down-regulates Cln3 translation and stability (9). CLN3 transcription is maintained throughout the cell cycle but displays some cell cycle periodicity that depends on early cell cycle boxes (ECB) sites upstream of the CLN3 promoter (10, 11). Glucose media induce CLN3 transcription and promote rapid G1 progression (12). Glucose induction of CLN3 requires glycolysis but not cell cycle progression and is not affected by mutations in the well characterized glucose repression or induction pathways (13, 14). Azf1, a glucose-activated transcription factor, binds to repeated elements in the CLN3 promoter (15, 16). Thus, CLN3 is an integrator for signals that regulate the rate of G1 progression in S. cerevisiae. The classical explanation for daughter cell G1 delay is the critical size model, in which daughter cells remain in G1 until they reach a cell size that triggers entry into S phase (17). In this model, the essential difference between mother and daughter is cell size. Shortly after this model was proposed, Wheals' group (18-20) published a set of studies indicating that the critical size model does not adequately explain yeast cell cycle control. Perhaps their most striking finding was a size-independent component of daughter cell G1 delay (18, 19). This size-independent G1 delay in daughters indicates that at least part of the daughter cell G1 delay is an intrinsic property of the daughter cell. More recent work has established several mechanisms that produce differential gene expression between mother and daughter cells. The HO gene is expressed exclusively in mother cells (21). ASH1 mRNA is transported into the growing bud and accumulates only in daughter cells, where the Ash1 protein functions to block HO expression (22-24). In addition to Ash1, the Ace2 transcription factor accumulates exclusively in daughter cells (25, 26), and Ace2 target genes are expressed only in daughters (25). These results, demonstrating a daughter-specific pattern of gene expression, provide a conceptual framework to explain the intrinsic G1 delay in daughter cells. We present work based on the hypothesis that daughter cell G1 delay depends on the specific localization of Ace2 to the daughter cell. In this model, daughter cell G1 delay would be determined not by cell size but by the asymmetric distribution of Ace2 between mother and daughter cells. We report that Ace2 delays G1 in daughter cells. Deletion of ACE2 produces daughter cells that proceed through G1 at the same rate as mother cells. In addition, an ACE2 (G128E) mutation that allows Ace2 to function in both mothers and daughters prolongs G1 in mothers, so that mother/daughter pairs proceed through G1 at the same rate. Ace2 acts as a negative regulator of CLN3 expression in daughter cells through an indirect mechanism.
ISSN
0027-8424
First Page
10275
Last Page
10280
Recommended Citation
Markwardt, David D.; Laabs, Tracy L.; Slattery, Matthew G.; Newcomb, Laura L.; Stillman, David J.; and Heideman, Warren, "ACE2 is required for daughter cell-specific G1 delay in Saccharomyces cerevisiae" (2003). Zoology Faculty Work. 34.
https://digitalcommons.owu.edu/zool_pubs/34
Link Out URL
https://doi.org/10.1073/pnas.1833999100